I am starting a project I'm calling "The Peripheral Levodopa Project".
Anyone with a brain is invited to help.
As you read below there are questions in RED, BOLD font. We need answers! Comment, email me, whatever. I need pertinent ideas, more questions, research papers, you name it. Read on. This is really important. There are anti-Parkinson's strategies just sitting there right in front of us.
Levodopa and Dopamine Circulation Throughout The Body
In reading about the kidneys’ production of dopamine, I came to realize that they produce dopamine from circulating levodopa; they do not produce their own levodopa. Where does all that levodopa come from I thought? It turns out that the mesenteric organs produce a large net amount of circulating levodopa.
https://academic.oup.com/jcem/article/82/11/3864/2866142
In fact, the brain is a minor levodopa producer when compared to the mesenteric organs. The article states:
"The existence of a peripheral DA system, independent of the sympathoadrenal system, is suggested by the considerable DA formed in the body and not converted to other catecholamines (11). Although originally thought to reflect DA formation in the central nervous system, it is now clear that the brain is a minor source of DA metabolites (12). The origins of the large amounts of DA produced elsewhere in the body are not established, but could reflect formation within a putative dopaminergic system in the digestive tract."
Further, the article goes on to provide the table below of results from people who were tested during abdominal surgery. The blood flows into and out of the mesenteric organs, the kidneys, and liver were tested for levodopa (DOPA) and dopamine (DA). The table indicates major net production of DOPA from the mesenteric organs, and major net consumption of DOPA by the kidneys and liver:
I thought I’d try to make a diagram of all this.
In the diagram below I put a throttle symbol to represent that some levodopa is removed before it reaches the brain by B6 which is converted to AADC (Aromatic Amino Acid Decarboxylase) and/or acts as a required cofactor to AADC. AADC converts the levodopa to dopamine, which mostly cannot make it through the blood brain barrier. Conversely B6 is removed from the system by the drug carbidopa. So in a mechanical representation, Since this is done in circulation and there’s no single organ that does it, this is like a throttle, like the accelerator pedal on your car. You step on the throttle and B6 dispatches to the muscles, and out of circulation so it’s no longer converting levodopa to dopamine, And the levodopa makes it into your brain. Synthetically, if you take the drug carbidopa it destroys the B6 temporarily until you eat again, but during that window there is no B6 and the levodopa makes it to the brain. However note that in this case the B6 doesn’t go to your muscles to do it’s job there. It’s just gone in this case. (I am working on finding the citations that show all assertions from this paragraph. here is one on my Google drive: https://drive.google.com/drive/folders/1--McOCvdem8hQjz8Twj49A49wLdRjnz3 )
Let off the accelerator and some of the B6 returns to circulation trimming back the levodopa that makes it to your brain.
So my thinking is that if we can improve the performance of the mesenteric organs and determine strategies to minimize circulating B6, we may be able to reduce or eliminate the need for medication.
Here’s my first attempt, comments appreciated:
The observations above suggest that if we can understand, operate, and manipulate B6 metabolism, we should be able to allow more of the body's natural levodopa production to enter the brain, and thereby reduce the dosages and the toll of medications for those with PD.
If we were to imagine a control panel for setting the body for admitting peripheral levodopa across the blood brain barrier unimpeded, it might look something like this:
Questions (and opportunities) Abound:
- How does the pancreas produce levodopa? How do we get it to produce more?
- The spleen is cited both as a mesenteric organ, and as part of the lymphatic system. Does the / how does the spleen produce levodopa? How do we get it to produce more?
-How do the colon, mesentery, and small intestine produce levodopa? How do we get them to produce more?
- How do we adjust liver metabolism away from destroying levodopa?
- How do we adjust kidney metabolism away from destroying levodopa? How do we slow the conversion of levodopa to dopamine in the adrenal cortices of the kidneys?
B6 Questions:
-What are the sources and sinks of B6?
-Which processes reversibly bind B6?
-Which processes irreversibly bind or destroy B6?
- Which processes take B6 out of circulation so that it cannot interact with AADC?
The general thought is that if we preoccupy healthy levels of B6 in other reversible processes, they won't interfere with levodopa entering the brain - and yet the B6 will be REVERSIBLY available when needed.
In order to understand vitamin B6 here is a good reference from the Linus Pauling Institute and OSU:
Here are the functions of B6 in nutshells (many):
B6 Functions
Vitamin B6 must be obtained from the diet because humans cannot synthesize it. PLP plays a vital role in the function of over 100 enzymes that catalyze essential chemical reactions in the human body (4). PLP-dependent enzymes have been classified into five structural classes known as Fold Type I-V (5):
- Fold Type I - aspartate aminotransferase family
- Fold Type II - tryptophan synthase family
- Fold Type III - alanine racemase family
- Fold Type IV - D-amino acid aminotransferase family
- Fold Type V - glycogen phosphorylase family
The many biochemical reactions catalyzed by PLP-dependent enzymes are involved in essential biological processes, such as hemoglobin and amino acid biosynthesis, as well as fatty acid metabolism. Of note, PLP also functions as a coenzyme for glycogen phosphorylase, an enzyme that catalyzes the release of glucose from stored glycogen. Much of the PLP in the human body is found in muscle bound to glycogen phosphorylase. PLP is also a coenzyme for reactions that generate glucose from amino acids, a process known as gluconeogenesis (6).
QUESTION: It is notable that 3, B6 molecules are bound to each glycogen phosphorylase molecule. What a MASSIVE B6 storage! Is this a reversible process.
QUESTION: It is notable that 3, B6 molecules are bound to each glycogen phosphorylase molecule. What a MASSIVE B6 storage! Is this a reversible process.
Nervous system function
In the brain, the PLP-dependent enzyme aromatic L-amino acid decarboxylase catalyzes the synthesis of two major neurotransmitters: serotonin from the amino acid tryptophan and dopamine from L-3,4-dihydroxyphenylalanine (L-Dopa). Other neurotransmitters, including glycine, D-serine, glutamate, histamine, and γ-aminobutyric acid (GABA), are also synthesized in reactions catalyzed by PLP-dependent enzymes (7).
Hemoglobin synthesis and function
PLP functions as a coenzyme of 5-aminolevulinic acid synthase, which is involved in the synthesis of heme, an iron-containing component of hemoglobin. Hemoglobin is found in red blood cells and is critical to their ability to transport oxygen throughout the body. Both pyridoxal and PLP are able to bind to the hemoglobin molecule and affect its ability to pick up and release oxygen. However, the impact of this on normal oxygen delivery to tissues is not known (6, 8). Vitamin B6 deficiency may impair hemoglobin synthesis and lead to microcytic anemia (3).
QUESTION: Since the spleen stores a massive amount of red blood cells, could we capitalize on spleen function to sequester B6?
QUESTION: Since the spleen stores a massive amount of red blood cells, could we capitalize on spleen function to sequester B6?
Tryptophan metabolism
Deficiency in another B vitamin, niacin, is easily prevented by adequate dietary intakes. The dietary requirement for niacin and the niacin coenzyme, nicotinamide adenine dinucleotide (NAD), can be also met, though to a fairly limited extent, by the catabolism of the essential amino acid tryptophan in the tryptophan-kynurenine pathway (Figure 2). Key reactions in this pathway are PLP-dependent; in particular, PLP is the cofactor for the enzyme kynureninase, which catalyzes the conversion of 3-hydroxykynurenine to 3-hydroxyanthranilic acid. A reduction in PLP availability appears to primarily affect kynureninase activity, limiting NAD production and leading to higher concentrations of kynurenine, 3-hydroxykynurenine, and xanthurenic acid in blood and urine (Figure 2) (9). Thus, while dietary vitamin B6 restriction impairs NAD synthesis from tryptophan, adequate PLP levels help maintain NAD formation from tryptophan (10). The effect of vitamin B6 inadequacy on immune activation and inflammation may be partly related to the role of PLP in the tryptophan-kynurenine metabolism (see Disease Prevention).
QUESTION: Turkey anyone?

Hormone function
Steroid hormones, such as estrogen and testosterone, exert their effects in the body by binding to steroid hormone receptors in the nucleus of target cells. The nuclear receptors themselves bind to specific regulatory sequences in DNA and alter the transcription of target genes. Experimental studies have uncovered a mechanism by which PLP may affect the activity of steroid receptors and decrease their effects on gene expression. It was found that PLP could interact with RIP140/NRIP1, a repressor of nuclear receptors known for its role in reproductive biology (11). Yet, additional research is needed to confirm that this interaction can enhance RIP140/NRIP1 repressive activity on steroid receptor-mediated gene expression. If the activity of steroid receptors for estrogen, progesterone, testosterone, or other steroid hormones can be inhibited by PLP, it is possible that vitamin B6 status may influence one's risk of developing diseases driven by steroid hormones, such as breast and prostate cancers (6).
Nucleic acid synthesis
The synthesis of nucleic acids from precursors thymidine and purines is dependent on folate coenzymes. The de novo thymidylate (dTMP) biosynthesis pathway involves three enzymes: dihydrofolate reductase (DHFR), serine hydroxymethyltransferase (SHMT), and thymidylate synthase (TYMS) (Figure 3). PLP serves as a coenzyme for SHMT, which catalyzes the simultaneous conversions of serine to glycine and tetrahydrofolate (THF) to 5,10-methylene THF. The latter molecule is the one-carbon donor for the generation of dTMP from dUMP by TYMS.
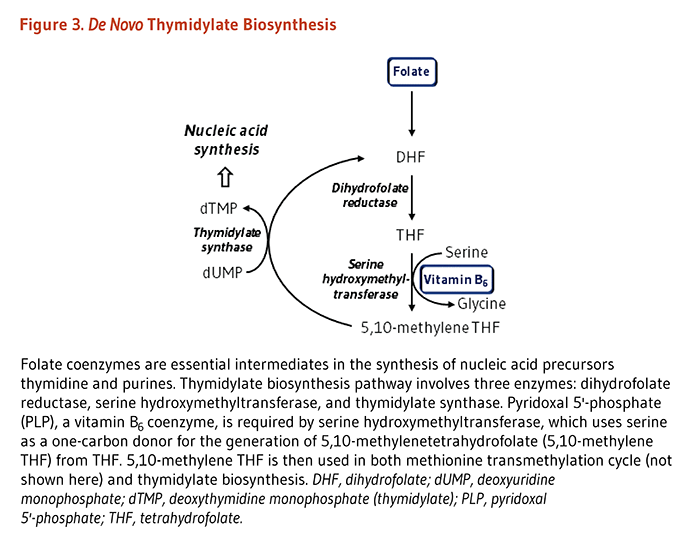
No comments:
Post a Comment